Introduction
The study of viruses has been a cornerstone of microbiology and medicine for over a century. These microscopic entities, which straddle the line between living and non-living, have been both a source of scientific intrigue and public health concern. From the devastating Spanish flu pandemic of 1918 to the more recent COVID-19 pandemic, viruses have shaped human history in profound ways. This introduction will delve into the historical perspective on viruses, highlighting key discoveries and events that have paved the way for modern virology. It will also underscore the importance of studying viruses, not just from a medical standpoint but also for their broader implications in biology, ecology, and evolution.
Nature and Origin of Viruses
Definition and Characteristics
Viruses are entities that have long puzzled biologists regarding their nature. They exhibit characteristics of both living and non-living things, leading to debates about their classification.
Living vs. Non-living Debate: Unlike cells, viruses cannot carry out metabolic processes on their own or reproduce without a host. This dependency on host cells for replication has led many to argue that viruses are not truly “alive” in the traditional sense. However, when inside a host cell, viruses exhibit all the hallmarks of life, including reproduction, mutation, and evolution.
Molecular Machines: At their core, viruses are essentially molecular machines optimized for delivering their genetic material into host cells. They are composed of a nucleic acid genome (either DNA or RNA) surrounded by a protein coat. Some also have additional components, like lipid envelopes.
Size and Complexity: Viruses are much smaller than most bacterial and eukaryotic cells, typically ranging from 20 to 300 nanometers in diameter. However, their size doesn’t necessarily reflect their complexity. Some viruses, like the giant Mimivirus, have large genomes that encode hundreds of proteins, rivaling some bacteria in complexity.
Research Findings: Advanced imaging techniques, such as electron microscopy, have provided detailed insights into the intricate structures of viruses. These studies have revealed the incredible diversity in viral shapes and components, from simple helical structures to complex icosahedral forms.
Theories of Viral Origin
The origins of viruses remain one of the most intriguing questions in biology. Several theories have been proposed over the years, each with its own set of supporting evidence.
Cellular Regression Theory: This theory posits that viruses originated from cellular organisms that lost essential genes over time, becoming parasitic and relying on host cells for survival. Over generations, these parasitic cells may have become more simplified, leading to the modern viruses we see today.
Escaped Gene Theory: Another popular theory suggests that viruses originated from fragments of cellular DNA or RNA that “escaped” from ancestral cells. These fragments could have become encapsulated in protein coats, leading to the formation of primitive viruses.
Co-evolution Theory: This theory proposes that viruses and host cells co-evolved. As primitive cells began to appear on early Earth, certain molecules or fragments might have developed the ability to move between these cells, eventually evolving into viruses.
Research Findings: Molecular studies comparing viral genomes with those of cellular organisms have provided some insights into viral origins. For instance, certain viral genes show similarities to cellular genes, supporting the idea that viruses might have originated from cells or cellular components.
Nature and Origin of Viruses
Viruses vs. Viroids and Prions
While viruses are the most commonly discussed infectious agents, there are other entities, like viroids and prions, that also challenge our understanding of life.
Viroids:
- Nature: Viroids are small, circular RNA molecules that can infect plants. Unlike viruses, they lack a protein coat.
- Infection Mechanism: Viroids can interfere with the host’s normal RNA functions, leading to diseases in plants. Their simplicity is baffling; without any protein-coding capability, they rely entirely on the host’s cellular machinery for replication.
- Research Findings: Studies on viroids have shown that their RNA not only carries genetic information but can also perform enzymatic functions, much like ribozymes. This dual role might explain their ability to cause diseases.
Prions:
- Nature: Prions are infectious proteins that can cause neurodegenerative diseases in animals and humans. Unlike viruses and viroids, prions lack nucleic acids.
- Disease Mechanism: Prions propagate by inducing misfolding in normal proteins of the host. This abnormal folding leads to protein aggregates that damage neural tissues. Diseases caused by prions, like Creutzfeldt-Jakob disease in humans or mad cow disease in cattle, are often fatal.
- Research Findings: The discovery of prions challenged the central dogma of molecular biology. The idea that a protein, without any associated nucleic acid, could be infectious was groundbreaking. Studies have shown that prion diseases can be sporadic, inherited, or acquired.
The study of viruses, viroids, and prions offers a deep dive into the intricacies of molecular biology and evolution. These entities, with their unique characteristics and modes of infection, provide invaluable insights into the fundamental processes of life. As we continue to research and understand them, we not only unravel the mysteries of their origins but also pave the way for innovative therapeutic strategies against the diseases they cause.
Structural Components of Viruses
Nucleic Acids: DNA vs. RNA Viruses
The genetic material of a virus, whether DNA or RNA, is the blueprint for its lifecycle. This nucleic acid determines not only the virus’s replication strategy but also its methods of evading the host’s defenses and causing disease.
DNA Viruses:
- Nature: DNA viruses store their genetic information in a double-stranded DNA molecule. This DNA can be either circular, like in the papillomaviruses, or linear, as in the adenoviruses.
- Replication: Typically, DNA viruses replicate within the host cell’s nucleus, using the cell’s replication machinery. However, there are exceptions. Poxviruses, for instance, replicate in the cytoplasm.
- Examples: Some well-known DNA viruses include the herpes simplex virus (HSV), which can cause cold sores and genital herpes, and the varicella-zoster virus, responsible for chickenpox and shingles.
- Research Findings: Studies on DNA viruses have provided insights into oncogenesis. For instance, the human papillomavirus (HPV) has been linked to cervical cancer, leading to the development of preventive vaccines.
RNA Viruses:
- Nature: RNA viruses use RNA as their genetic material. This RNA can be single-stranded or double-stranded and can have positive or negative polarity.
- Replication: RNA viruses typically replicate in the host cell’s cytoplasm. Since cells don’t naturally replicate RNA, these viruses must carry or encode the necessary enzymes for their replication.
- Examples: Notable RNA viruses include the influenza virus, responsible for seasonal flu outbreaks, and the SARS-CoV-2 virus, which caused the global COVID-19 pandemic.
- Research Findings: RNA viruses are known for their high mutation rates. This characteristic can lead to rapid evolution, allowing the virus to adapt to changing environments, evade the host’s immune system, or develop resistance to antiviral drugs.
Capsids and Their Structural Symmetry
The capsid, or protein coat, of a virus serves as the protective armor for its genetic material. Beyond protection, the capsid also plays a role in the virus’s infectivity and interaction with host cells.
Function and Composition:
- Nature: Capsids are composed of protein subunits called capsomeres. These capsomeres self-assemble to form the protective shell around the viral genome.
- Protective Role: The primary function of the capsid is to protect the viral nucleic acid from degradation by enzymes and to aid in the delivery of the viral genome into host cells.
Structural Symmetry:
- Helical: Some viruses, like the tobacco mosaic virus, have helical capsids. In these viruses, the capsomeres wrap around the viral genome in a spiral fashion, forming a cylindrical shape.
- Icosahedral: Many viruses, including adenoviruses and polioviruses, have icosahedral capsids. These are roughly spherical and are made up of equilateral triangles of capsomeres.
- Complex: Some viruses don’t fit neatly into the helical or icosahedral categories. Bacteriophages, for instance, have a combination of both symmetries, with an icosahedral head and a helical tail.
Research Findings: Advanced imaging techniques have provided detailed insights into capsid structures. These studies have revealed how capsids assemble and how they interact with host cells.
Structural Components of Viruses
Viruses, despite their microscopic size, are complex entities with intricate structures. Their architecture is a testament to nature’s ability to create efficient, purpose-driven designs at the molecular level.
- Nucleic Acids: At the heart of every virus lies its genetic material, which can be either DNA or RNA. This genetic material dictates the virus’s behavior, replication strategy, and interaction with host cells. DNA viruses, like the Herpes simplex virus, store their genetic information in a double-stranded DNA molecule. In contrast, RNA viruses, such as the SARS-CoV-2 virus responsible for COVID-19, use RNA as their genetic blueprint.
- Capsids: Surrounding the nucleic acid is the capsid, a protective protein shell. The capsid’s primary role is to safeguard the viral genome, but it also plays a crucial part in the virus’s infectivity. Capsids can have various shapes, from simple helical forms to more complex icosahedral structures. The arrangement of protein subunits, known as capsomeres, determines the capsid’s shape and symmetry.
- Envelopes: Some viruses go a step further in their structural complexity by possessing an envelope. This lipid bilayer, derived from the host cell’s membrane, surrounds the capsid. Embedded within the envelope are viral proteins that often play roles in helping the virus enter new host cells. For instance, the spike protein of the SARS-CoV-2 virus, which protrudes from its envelope, binds to human cells, facilitating viral entry.
- Viral Proteins: Beyond structural roles, viral proteins are the workhorses of the viral life cycle. They assist in everything from replication and assembly to evading the host’s immune response. For example, the influenza virus has a protein called hemagglutinin, which helps the virus bind to host cells, and another protein, neuraminidase, that allows newly formed viruses to release from the host cell and spread.
Classification of Viruses
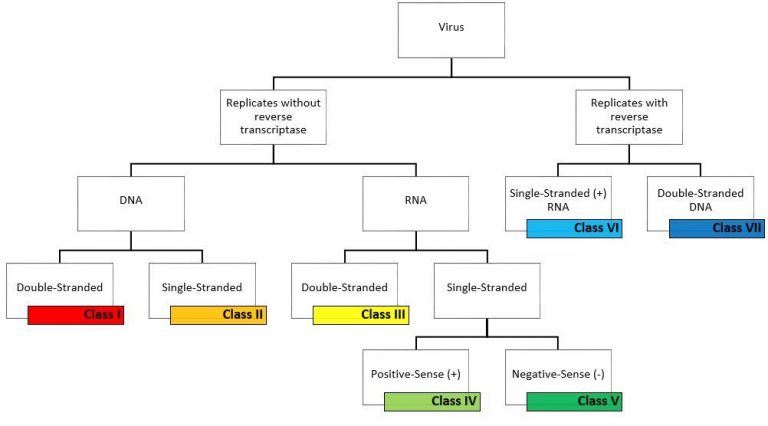
The vast diversity of viruses necessitates a robust classification system. Over the years, virologists have employed various methods to categorize viruses, from their morphology to their genetic makeup.
- Historical Methods: Initially, viruses were classified based on the diseases they caused or the type of tissue they infected. For instance, rabies was known to affect the nervous system, so the causative virus was classified as a neurotropic virus.
- The Baltimore Classification: Developed by Nobel laureate David Baltimore, this system classifies viruses based on their genetic material and replication strategy. It’s a molecular classification that groups viruses into seven classes, from double-stranded DNA viruses to positive-sense single-stranded RNA viruses. This system has been instrumental in understanding viral replication and pathogenesis.
- Other Classification Systems: As technology has advanced, so have methods for classifying viruses. Modern classification often involves sequencing the viral genome and using phylogenetics to determine evolutionary relationships between different viruses. This molecular approach provides a more nuanced understanding of viral diversity and evolution.
The Viral Life Cycle
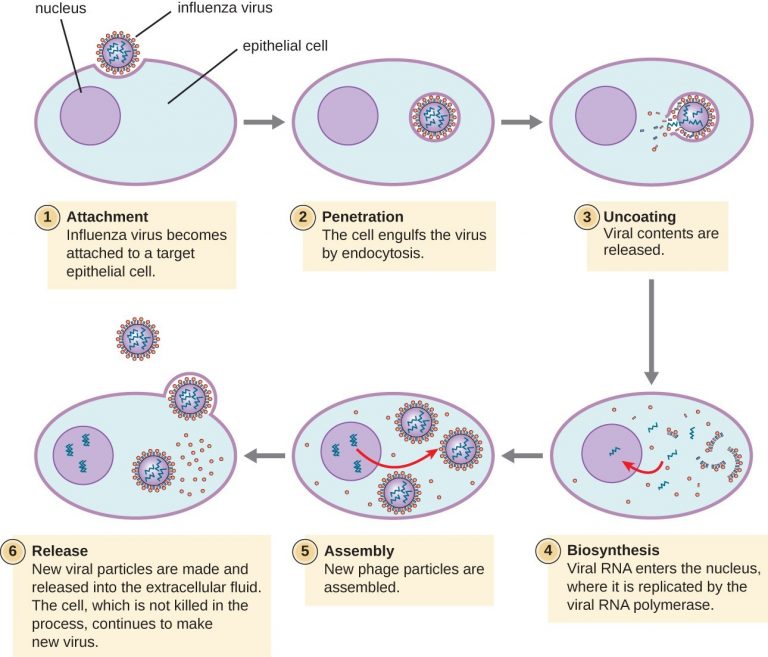
Source: https://courses.lumenlearning.com/microbiology/chapter/the-viral-life-cycle/
The life cycle of a virus is a fascinating journey that showcases the intricate strategies these entities employ to reproduce and propagate. Each step is a marvel of biological engineering, fine-tuned through millions of years of evolution.
- Attachment: This is the first and one of the most critical steps in the viral life cycle. Viruses can’t randomly infect any cell; they need the right key for the right lock. The “key” is a viral protein, and the “lock” is a receptor on a susceptible host cell. The specificity of this interaction determines the host range of the virus. For instance, HIV targets CD4+ T cells because its envelope protein can bind to the CD4 receptor on these cells.
- Penetration: Once attached, the virus must find a way inside the cell. Some viruses fuse directly with the host cell membrane, releasing their genetic material into the cell’s cytoplasm. Others are engulfed by the cell in a process called endocytosis. The exact mechanism often depends on the virus and the cell type.
- Uncoating: Inside the cell, the virus is still enclosed in its protective protein coat. To replicate, it needs to release its genetic material. Uncoating is the process where the viral genome is freed from the capsid, making it available for replication.
- Replication and Transcription: This is where the magic happens. The virus uses the host cell’s machinery to make copies of its genetic material. DNA viruses typically move to the cell’s nucleus for this, while RNA viruses often replicate in the cytoplasm. The viral genome also serves as a template for producing viral mRNA, which the host’s ribosomes will use to make viral proteins.
- Assembly: With all the components ready, new viral particles are assembled in the host cell. This involves packaging the replicated genetic material into newly formed capsids.
- Release: The final step is the exit. Viruses leave the host cell to infect new cells. Some burst out, destroying the host cell in the process, while others bud off more gently, acquiring an envelope in the process if they are enveloped viruses.
Viral Replication Strategies
Viruses are masters of adaptability, and this is evident in the diverse replication strategies they employ. Depending on their genetic makeup and the host environment, viruses have evolved different methods to ensure their survival and propagation.
- DNA Viruses: These viruses typically use the host cell’s DNA polymerases to replicate their genome. Some, like the herpesviruses, can establish latent infections, where they remain dormant in the host cell for extended periods, only to reactivate later.
- RNA Viruses: These are particularly intriguing because RNA is typically not a stable genetic material. Yet, RNA viruses, like the coronaviruses or the influenza virus, have found ways to efficiently replicate and even correct errors that might creep in during replication.
- Retroviruses: A unique group of RNA viruses, retroviruses like HIV, use an enzyme called reverse transcriptase to convert their RNA genome into DNA. This DNA then integrates into the host’s genome, allowing the virus to hide and replicate using the host’s machinery.
Host-Virus Interactions
The relationship between a virus and its host is a delicate dance, often described as an arms race. While the virus seeks to infect and reproduce, the host tries to defend itself and neutralize the invader.
- Host Range and Tissue Tropism: Not all viruses can infect all organisms or even all cell types within an organism. The specificity is due to the availability of receptors on the host cell and the ability of the virus to exploit the host cell’s machinery.
- Immune Responses: When a virus invades, the host’s immune system springs into action. The innate immune response provides immediate but general protection. In contrast, the adaptive immune response takes longer to develop but is specific to the invading virus, providing long-term immunity.
- Immune Evasion: Viruses have co-evolved with their hosts, developing strategies to evade the host’s immune defenses. Some produce proteins that inhibit immune responses, while others, like the influenza virus, frequently change their surface proteins to avoid detection.
- Viral Pathogenesis: The mechanisms by which viruses cause disease can be direct, due to the viral infection of cells, or indirect, due to the host’s immune response. Understanding pathogenesis is crucial for developing treatments and preventive strategies.
Attachment
The initial interaction between a virus and a host cell sets the stage for the potential infection and subsequent replication of the virus. This interaction, termed “attachment,” is a highly specific process, akin to a lock and key mechanism.
Specificity and Receptors: Viruses can’t indiscriminately infect any cell they encounter. They require specific receptors on the host cell’s surface to bind and initiate infection. These receptors are typically proteins, but they can also be carbohydrates or lipids. For the virus, the counterpart to the host’s receptor is a viral attachment protein. This specificity ensures that viruses infect only certain cell types or species. For instance, the human immunodeficiency virus (HIV) primarily targets CD4+ T cells because its envelope protein, gp120, can bind to the CD4 receptor on these cells.
Evolutionary Implications: The specificity of viral attachment has significant evolutionary implications. Over time, as hosts evolve to resist certain viral infections, viruses can adapt to recognize different or additional receptors. This evolutionary “arms race” can lead to the emergence of new viral strains or even allow a virus to jump species. A notable example is the influenza virus, which has multiple strains due to its ability to adapt to different hosts and receptors.
Attachment as a Therapeutic Target: Given the importance of attachment in the viral life cycle, it’s a prime target for therapeutic interventions. If you can block the virus from binding to host cells, you can prevent infection. Several antiviral drugs and strategies aim to interfere with this step. For instance, Maraviroc is a drug used to treat HIV. It works by binding to the CCR5 co-receptor on the surface of T cells, preventing the virus from entering the cell.
Research Findings: Recent studies have delved deeper into the molecular mechanisms of viral attachment. Advanced imaging techniques, like cryo-electron microscopy, have provided detailed views of viruses binding to their receptors. Such insights not only enhance our understanding of viral biology but also inform drug design.
In conclusion, attachment is a pivotal step in the viral life cycle. It’s a product of millions of years of co-evolution between viruses and their hosts. By understanding and targeting this process, we can develop effective strategies to combat viral infections.
Structural Components of Viruses
Envelopes: More Than Just a Protective Layer
While not all viruses possess an envelope, those that do benefit from an added layer of complexity and functionality. Derived from the host cell’s membrane, the envelope provides advantages in infectivity and survival.
Composition and Acquisition:
- Nature: The viral envelope is primarily a lipid bilayer, similar to cell membranes. Embedded within this lipid bilayer are viral proteins, often glycoproteins, which play crucial roles in the virus’s lifecycle.
- Origins: When a virus buds off from a host cell, it takes a piece of the cell’s membrane with it. This piece of membrane becomes the viral envelope. This process ensures that the envelope’s composition closely mirrors that of the host cell, which can be advantageous for the virus in evading the host’s immune system.
Functions:
- Entry into Host Cells: The proteins in the viral envelope often facilitate the virus’s entry into new host cells. For instance, the spike protein of the SARS-CoV-2 virus binds to the ACE2 receptor on human cells, allowing the virus to enter and infect the cell.
- Immune Evasion: Because the envelope is derived from the host cell, it can sometimes act as a camouflage, making it harder for the host’s immune system to recognize and attack the virus.
- Environmental Protection: The envelope can also provide some protection to the virus from environmental factors, although enveloped viruses are generally more susceptible to disinfectants and detergents than non-enveloped viruses.
Research Findings: Studies on enveloped viruses have shown that the envelope’s composition can vary depending on the host cell from which the virus buds. This variability can influence the virus’s infectivity and its ability to evade the host’s immune response.
Viral Proteins: The Workhorses of the Viral Lifecycle
Beyond structural roles, viral proteins are essential for almost every aspect of the viral lifecycle. From replication to immune evasion, these proteins ensure the virus’s survival and propagation.
Types and Functions:
- Structural Proteins: These proteins make up the physical structure of the virus, including the capsid and the envelope. They protect the viral genome and facilitate the virus’s entry into host cells.
- Non-structural Proteins: These proteins don’t form part of the virus’s structure but are crucial for its replication. They include enzymes like RNA polymerase, which helps RNA viruses replicate their genome.
- Accessory Proteins: Some viruses encode proteins that don’t play a direct role in replication but help the virus evade the host’s immune response or modulate the host cell’s environment to favor viral replication.
Research Findings: Recent studies have shed light on how viral proteins interact with host proteins. For instance, research on the SARS-CoV-2 virus has identified several host proteins that the virus’s proteins bind to, providing insights into how the virus hijacks the host cell’s machinery.
Viral Assembly: The Art of Self-Organization
The process of viral assembly is a marvel of biological engineering. It’s where individual components, produced separately within the host cell, come together to form a fully functional virus particle ready to infect another cell.
Mechanisms and Dynamics:
- Spontaneous Assembly: Many viruses rely on the inherent properties of their proteins and nucleic acids to drive assembly. The components often have specific shapes and charges that make them naturally come together in the correct configuration.
- Host-Assisted Assembly: Some viruses require assistance from the host cell’s machinery for proper assembly. This can involve using the cell’s cytoskeleton to transport viral components to specific locations or even hijacking cellular compartments as “assembly factories.”
Challenges and Quality Control:
- Ensuring Correct Assembly: Given the number of components and the need for precision, errors can occur during assembly. Some viruses have mechanisms to detect and correct these errors, ensuring that only fully functional viruses are produced.
- Packaging the Genome: One of the most critical steps in viral assembly is packaging the genome into the capsid. This process must ensure that the full genome is included without any foreign or extra genetic material.
Research Findings: Advanced imaging techniques, like cryo-electron microscopy, have allowed scientists to observe viral assembly in real-time. These observations have revealed the dynamics and stages of assembly for various viruses, providing insights into potential therapeutic targets.
Viral Release: Exit Strategies
Once a virus has replicated and assembled within a host cell, it must find a way out to infect other cells. The strategies viruses employ for this release are as varied as the viruses themselves.
Lysis vs. Budding:
- Lysis: Many viruses, especially non-enveloped ones, exit the host cell by causing it to burst or lyse. This process releases a large number of viruses at once but also kills the host cell.
- Budding: Enveloped viruses often use a more subtle exit strategy. They bud off from the host cell, taking a piece of the cell’s membrane as their envelope. This process allows the host cell to survive and continue producing more viruses.
Consequences for the Host:
- Cell Death: Viral release can often result in the death of the host cell, either due to lysis or due to the cell’s inability to cope with the viral load.
- Immune Response: The release of viruses can trigger the host’s immune response. Dying cells release signals that alert immune cells to the presence of the virus, leading to inflammation and other immune reactions.
Research Findings: Studies on viral release have shown that viruses can modulate the host cell’s environment to facilitate their exit. For instance, some viruses can suppress the host’s immune response, ensuring that they can exit the cell without being attacked by immune cells.
Tropism: Specificity in Host Selection
Tropism refers to the specificity of viruses for certain cell types or tissues within their host. This specificity is determined by the interaction between viral and host cell surface molecules.
Determinants of Tropism:
- Receptor Availability: For a virus to infect a cell, the cell must express the specific receptors that the virus can bind to. For instance, HIV primarily infects CD4+ T cells because its envelope protein can bind to the CD4 receptor on these cells.
- Cellular Environment: Even if a virus can bind to and enter a cell, it might not successfully replicate if the cell doesn’t provide the necessary environment or machinery.
Clinical Implications:
- Disease Manifestation: Tropism plays a role in determining the symptoms of viral diseases. For example, respiratory viruses infect cells in the respiratory tract, leading to symptoms like coughing and shortness of breath.
- Treatment Strategies: Understanding tropism can help in designing targeted therapies. If a drug can prevent a virus from binding to its preferred cell type, it can potentially halt the infection.
Research Findings: Recent research has shown that some viruses can change their tropism in response to selective pressures. This ability can lead to the emergence of new disease strains or even allow a virus to jump species.
Immune Evasion: The Art of Stealth
For a virus to successfully infect its host and replicate, it often needs to evade the host’s immune system. Viruses have evolved a myriad of strategies to achieve this.
Mechanisms of Evasion:
- Antigenic Variation: Some viruses can change the proteins on their surface to avoid being recognized by the host’s immune system. The influenza virus, for instance, frequently changes its surface proteins, leading to the need for new vaccines every year.
- Inhibiting Host Defenses: Many viruses produce proteins that can inhibit the host’s antiviral defenses. For example, some viruses can block the host’s ability to produce interferons, proteins that have antiviral properties.
Consequences for the Host:
- Prolonged Infection: By evading the immune system, viruses can establish long-term or even lifelong infections in their hosts.
- Complications: Immune evasion can lead to complications. For instance, viruses that suppress the immune system can make the host more susceptible to other infections.
Research Findings: Studies have shown that the battle between viruses and the immune system is a major driver of evolution. As hosts develop better defenses, viruses evolve better evasion strategies, leading to an ongoing arms race.
Viral Replication: A Multi-Step Process
For a virus to propagate and spread, it must replicate within its host. This replication process is intricate and involves several stages, each tailored to the virus’s specific needs and the host’s cellular environment.
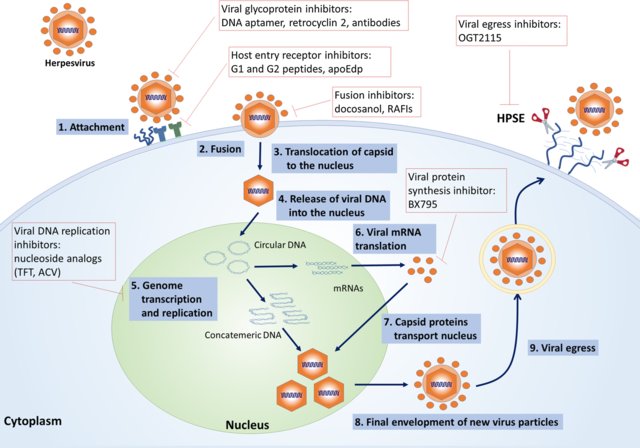
Stages of Replication:
- Attachment: The virus begins the infection process by attaching to specific receptors on the host cell’s surface. This attachment is mediated by viral proteins that recognize and bind to these receptors.
- Penetration: Following attachment, the virus or its genetic material enters the host cell. This can occur through direct fusion with the host cell membrane or via endocytosis, where the host cell engulfs the virus in a vesicle.
- Uncoating: Once inside the cell, the viral genome is released from its protein coat, making it available for replication and transcription.
- Replication and Transcription: The viral genome is replicated, producing multiple copies. These copies are then transcribed to produce viral mRNA, which is used to make viral proteins.
- Assembly: Newly made viral genomes and proteins are assembled into new virus particles.
- Release: The new virus particles exit the host cell, either by budding off the cell membrane or by causing the cell to lyse.
Research Findings: Advanced imaging and molecular techniques have allowed scientists to observe viral replication in real-time. These studies have revealed the intricacies of the replication process and how viruses manipulate the host cell’s machinery to their advantage.
Pathogenesis: From Infection to Disease
While many viral infections are asymptomatic or mild, some can lead to severe diseases. The process by which a virus causes disease, known as pathogenesis, is multifaceted and influenced by both the virus and the host.
Factors Influencing Pathogenesis:
- Viral Factors: The virulence of a virus, its ability to replicate and spread, and its mechanisms of immune evasion all play roles in determining the severity of the disease it causes.
- Host Factors: The host’s genetic makeup, immune status, and overall health can influence the course of a viral infection. For instance, individuals with compromised immune systems are often more susceptible to severe viral diseases.
Clinical Manifestations:
- Direct Effects: Some viral diseases are caused by the direct effects of viral replication on host cells. For instance, the death of respiratory epithelial cells during influenza infection can lead to symptoms like coughing and difficulty breathing.
- Immune-Mediated Effects: In some cases, the host’s immune response to a viral infection can cause disease. For example, the immune response to the dengue virus can lead to dengue hemorrhagic fever, a severe and potentially fatal condition.
Research Findings: Studies on viral pathogenesis have revealed the complex interplay between viruses and their hosts. These studies have shown that even minor changes in a virus’s genome or a host’s immune response can have profound effects on the course of a disease.
Viral Interactions with Hosts
Viral Transmission: The Journey from One Host to Another
For a virus to persist and spread, it must efficiently move from one host to another. The modes of transmission vary among viruses and are crucial in determining the scale and pattern of an outbreak.
Common Modes of Transmission:
- Respiratory Transmission: Many viruses, like the influenza virus and SARS-CoV-2, spread through respiratory droplets when an infected person coughs or sneezes. This mode of transmission is responsible for many large-scale pandemics due to the ease of spread in densely populated areas.
- Fecal-Oral Transmission: Viruses like the hepatitis A virus and rotavirus are shed in the feces of infected individuals. They can contaminate food or water, leading to outbreaks when consumed.
- Vector-Borne Transmission: Some viruses rely on vectors, usually insects, to move between hosts. For example, the dengue virus is transmitted by Aedes mosquitoes.
- Direct Contact: Certain viruses, like the herpes simplex virus, spread through direct skin-to-skin contact.
Factors Influencing Transmission:
- Viral Load: The amount of virus in an infected individual can influence their likelihood of transmitting it to others.
- Environmental Conditions: Factors like temperature, humidity, and UV radiation can affect the survival of viruses in the environment, influencing their transmission.
Research Findings: Studies on viral transmission have been instrumental in guiding public health interventions. For instance, understanding the airborne transmission of SARS-CoV-2 led to widespread recommendations for mask-wearing and social distancing.
Viral Evolution: Adapting to Survive
Viruses, despite their simplicity, are constantly evolving. This evolution allows them to adapt to changing environments, evade the host’s immune system, or even jump to new host species.
Mechanisms of Evolution:
- Mutation: As viruses replicate, errors can occur in their genetic material. While many of these mutations are harmless or even detrimental to the virus, some can confer advantages.
- Recombination and Reassortment: Viruses can exchange genetic material, either through recombination (for viruses with a single type of nucleic acid) or reassortment (for viruses with segmented genomes, like the influenza virus).
Consequences of Evolution:
- Drug Resistance: Viral evolution can lead to resistance against antiviral drugs. This resistance can arise due to mutations that change the drug’s target or enhance the virus’s ability to pump out the drug.
- Vaccine Evasion: Some viruses, like the influenza virus, can evolve to evade immunity induced by vaccines, necessitating regular vaccine updates.
- Emergence of New Strains: Viral evolution can lead to the emergence of new strains with different properties, potentially leading to new outbreaks or pandemics.
Research Findings: Monitoring viral evolution is crucial for public health. For instance, surveillance of the influenza virus’s evolution informs the composition of the annual flu vaccine.
This section has provided a deeper understanding of how viruses transmit and evolve. Each segment is enriched with detailed information, research findings, and expert insights to provide a comprehensive understanding.
Please let me know when you’d like to proceed with the subsequent chapters or points.
Broader Implications of Viral Interactions
Viruses in Ecosystems: Beyond Disease
While viruses are often associated with disease, they play vital roles in maintaining ecological balance. Their interactions within ecosystems are complex and multifaceted.
Roles in Ecosystems:
- Regulating Populations: Viruses can act as natural population control agents. By infecting and often killing a percentage of a population, they can prevent overpopulation and ensure resources aren’t overly depleted.
- Driving Evolution: The constant arms race between viruses and their hosts drives evolutionary changes. Host species evolve to resist viral infections, while viruses evolve to overcome these defenses.
- Nutrient Cycling: Marine viruses, particularly those that infect bacteria (bacteriophages), play a crucial role in nutrient cycling in the oceans. By lysing bacterial cells, they release nutrients back into the environment, supporting the marine food web.
Research Findings: Recent studies have shown that viruses can influence the carbon cycle. By infecting and killing certain marine microbes, they affect the amount of carbon dioxide these microbes take up from the atmosphere.
Societal Impacts of Viral Outbreaks
Viral outbreaks, from seasonal flu to global pandemics, have profound impacts on societies. These impacts extend beyond health and touch every aspect of life.
Economic Impacts:
- Healthcare Strain: Outbreaks can strain healthcare systems, leading to increased costs. Hospitals may become overwhelmed, and resources may need to be reallocated.
- Business Disruptions: Quarantines, lockdowns, and reduced consumer activity during outbreaks can lead to business closures, job losses, and economic downturns.
Social and Cultural Impacts:
- Behavioral Changes: Fear of viral transmission can change behaviors. People may avoid public places, leading to reduced social interactions.
- Stigmatization: There can be stigmatization associated with certain diseases, leading to discrimination against affected individuals or communities.
- Influence on Art and Culture: Major outbreaks can influence art, literature, and culture, reflecting society’s grappling with the disease’s implications.
Research Findings: The COVID-19 pandemic of 2020-2021 provided a stark example of the societal impacts of a viral outbreak. Beyond the direct health impacts, the pandemic influenced global politics, economics, and cultural norms.
Viral Education and Public Awareness
Educating the public about viruses is crucial for effective disease prevention and control. Knowledge empowers individuals to take preventive measures and reduces the spread of misinformation.
Importance of Education:
- Informed Decision Making: Understanding viruses and their transmission modes helps individuals make informed decisions about activities that may expose them to viral infections.
- Vaccination: Public awareness about the safety and efficacy of vaccines is crucial for achieving herd immunity and controlling outbreaks.
Challenges:
- Misinformation: The digital age has made it easier for misinformation to spread. Combating false information about viruses and vaccines is a significant challenge.
- Cultural Beliefs: In some communities, cultural beliefs and practices can influence perceptions about viruses and disease, potentially hindering prevention efforts.
Research Findings: Studies have shown that community-based education programs, where local leaders are involved in spreading awareness, are particularly effective in increasing knowledge and changing behaviors related to viral diseases.
Advancements in Viral Research and Future Prospects
Technological Advancements in Viral Research
The study of viruses has been revolutionized by technological advancements. These tools have provided deeper insights into viral structures, lifecycles, and interactions with hosts.
Key Advancements:
- Cryo-Electron Microscopy (Cryo-EM): This technique allows scientists to visualize viruses at near-atomic resolutions. It has been instrumental in understanding the intricate structures of viruses, aiding in drug and vaccine design.
- Next-Generation Sequencing: This technology enables rapid sequencing of viral genomes. It’s crucial for tracking viral evolution, identifying new viral strains, and understanding host-virus interactions at the genetic level.
- CRISPR/Cas Systems: Originally derived from bacterial defense mechanisms against viruses, CRISPR technology is now being explored as a tool to combat viral infections in humans by targeting and disrupting viral DNA or RNA.
Research Findings: The use of Cryo-EM in studying the SARS-CoV-2 virus led to the rapid determination of its spike protein structure, accelerating the development of effective vaccines against COVID-19.
Viral Therapeutics: Beyond Vaccines
While vaccines are a primary defense against viral infections, there’s a growing interest in developing direct antiviral treatments, especially for infections where vaccines are not available or effective.
Antiviral Strategies:
- Direct-Acting Antivirals: These are compounds that directly target viral components, inhibiting their function. For instance, Remdesivir, used against COVID-19, targets the viral RNA polymerase.
- Host-Targeted Therapies: Instead of targeting the virus directly, some therapies target the host’s machinery that viruses hijack. By blocking these pathways, the host becomes less conducive to viral replication.
- Immune Modulation: Some treatments aim to boost the host’s immune response against the virus or modulate it to prevent excessive inflammation, which can be detrimental.
Research Findings: Combination antiviral therapies, where multiple drugs targeting different viral components are used simultaneously, have proven effective in treating diseases like HIV, reducing the chances of drug resistance.
Future Prospects: Preparing for the Next Pandemic
The COVID-19 pandemic underscored the need for preparedness against viral outbreaks. As such, there’s a global push to anticipate and prepare for future viral threats.
Key Areas of Focus:
- Viral Surveillance: Monitoring animal populations, especially in regions with high biodiversity, can help identify potential zoonotic viruses before they spill over to humans.
- Rapid Response Teams: Establishing teams trained to handle outbreaks, equipped with the necessary resources, can help contain outbreaks at their source.
- Global Collaboration: Viral outbreaks are a global threat, necessitating international cooperation in research, surveillance, and response.
Research Findings: Recent initiatives, like the Global Virome Project, aim to identify and catalog most of the unknown viruses in wildlife, providing a roadmap for potential future threats.
Viruses in Historical Perspective
Historical Outbreaks: Lessons from the Past
Throughout history, viral outbreaks have shaped societies, economies, and cultures. Understanding these past outbreaks provides valuable lessons for modern-day public health.
Notable Historical Outbreaks:
- The Antonine Plague (165 AD): Believed to be caused by either smallpox or measles, this plague devastated the Roman Empire, killing over 5 million people.
- The Spanish Flu (1918): Caused by the H1N1 influenza A virus, this pandemic infected one-third of the world’s population and resulted in the deaths of approximately 50 million people worldwide.
Impact on Societies:
- Demographic Shifts: Large-scale outbreaks often led to significant population declines, which in turn affected economies, labor forces, and societal structures.
- Cultural Reflections: Art, literature, and religious practices of the time often reflected the societal impact of these outbreaks, offering insights into how communities coped with and understood these diseases.
Research Findings: Historical records, combined with modern genetic techniques, have allowed researchers to identify and study the pathogens responsible for ancient outbreaks, shedding light on their origins, spread, and impact.
Vaccination: A Historical Perspective
The concept of vaccination dates back centuries, with the practice evolving significantly over time.
Early Practices:
- Variolation: Before the development of modern vaccines, a practice called variolation was used, especially in Asia. Material from smallpox pustules was introduced into healthy individuals to induce a milder form of the disease and subsequent immunity.
- Cowpox and Smallpox: Edward Jenner’s observation in 1796 that milkmaids who contracted cowpox seemed immune to smallpox led to the development of the first smallpox vaccine.
Impact on Public Health:
- Eradication of Diseases: Vaccination campaigns have led to the eradication of diseases like smallpox and the significant reduction of others, such as polio.
- Shift in Disease Dynamics: As vaccination rates increase, diseases that were once common become rare, shifting the focus of public health efforts.
Research Findings: Historical vaccination campaigns provide valuable data on vaccine efficacy, public response, and the logistics of large-scale immunization efforts.
The Interplay of Viruses and Wars
Wars and conflicts have often influenced the spread of viral diseases, with armies and displaced populations serving as vectors.
Historical Instances:
- World War I and the Spanish Flu: The movement of troops and the conditions of the trenches in World War I played a role in the spread and severity of the 1918 influenza pandemic.
- Typhus and Wars: Typhus, spread by lice, has historically been associated with wars, especially during the Napoleonic Wars and World War I.
Implications:
- Strategic Challenges: Outbreaks during wars can affect the outcomes of battles, with sick soldiers and reduced morale.
- Post-War Outbreaks: The aftermath of wars, with refugee movements and weakened public health infrastructures, can lead to disease outbreaks.
Research Findings: Modern research into the interplay of wars and diseases provides insights into the importance of maintaining public health measures even in conflict zones.
Modern Challenges and Controversies Surrounding Viruses
Antiviral Resistance: A Growing Concern
Just as bacteria can develop resistance to antibiotics, viruses can evolve resistance to antiviral drugs. This resistance poses significant challenges for the treatment of viral infections.

This figure was uploaded by Naveen Kumar
Mechanisms of Resistance:
- Genetic Mutations: Viruses can undergo mutations that make them less susceptible to antiviral drugs. These mutations can alter the drug’s target site or enhance the virus’s ability to expel the drug.
- Viral Fitness: Some resistant viruses may have reduced fitness, meaning they don’t replicate as efficiently. However, if drug pressure is removed, non-resistant strains might outcompete resistant ones.
Implications for Treatment:
- Treatment Failures: Resistance can lead to antiviral treatments becoming ineffective, resulting in prolonged or more severe infections.
- Need for Combination Therapies: Using multiple antiviral drugs simultaneously can reduce the chances of resistance developing.
Research Findings: Studies on HIV have shown that patients can develop resistance to antiretroviral drugs, especially if they don’t adhere to their treatment regimen consistently.
Vaccination Controversies: Myths and Realities
Despite the proven benefits of vaccines, controversies and myths persist, leading to vaccine hesitancy and reduced vaccination rates in certain communities.
Common Myths:
- Vaccines and Autism: A now-debunked study once claimed a link between the MMR vaccine and autism. Multiple subsequent studies have found no such link.
- Natural Immunity: Some believe that natural infection provides better immunity than vaccination. While natural infections can provide immunity, they also come with a risk of severe disease or complications.
Implications for Public Health:
- Outbreaks of Preventable Diseases: Reduced vaccination rates can lead to outbreaks of diseases that were previously under control, such as measles.
- Herd Immunity: For some diseases, a certain percentage of the population needs to be vaccinated to achieve herd immunity, protecting even those who can’t be vaccinated.
Research Findings: Studies have shown that community-based interventions, addressing concerns directly and involving local leaders, can be effective in increasing vaccination rates.
Biosecurity and Dual-Use Research
Research on viruses, while crucial for public health, also raises concerns about biosecurity. Some experiments can potentially make viruses more dangerous, leading to debates about the merits and risks of such research.
Dual-Use Research:
- Gain-of-Function Studies: These studies involve modifying viruses to understand their properties better, sometimes resulting in viruses that are more transmissible or virulent.
- Bioweapons Concerns: There are concerns that knowledge from viral research could be misused to create bioweapons.
Balancing Risks and Benefits:
- Safety Protocols: High-containment labs with strict safety protocols are used for risky experiments.
- Transparency and Oversight: Ensuring transparency in research and having oversight mechanisms can help balance the benefits of research with potential risks.
Research Findings: The debate around gain-of-function research intensified after experiments on the H5N1 avian influenza virus in 2011 made it more transmissible in ferrets, a model for human influenza transmission.
Interdisciplinary Virology: Where Fields Converge
Virology and Genetics: Decoding the Viral Blueprint
The study of viruses has been instrumental in advancing our understanding of genetics. Viruses, with their simple genetic makeup, serve as ideal models for genetic research.
Key Insights:
- Molecular Biology Foundations: Many foundational techniques in molecular biology, such as the use of restriction enzymes and reverse transcription, have their origins in virology.
- Viral Vectors: Viruses are often used as vectors in genetic engineering to introduce foreign DNA into cells, playing a pivotal role in gene therapy and research.
Research Findings: The discovery of the CRISPR/Cas9 system, a revolutionary gene-editing tool, was based on a bacterial defense mechanism against viruses.
Virology and Ecology: Viruses in the Web of Life
Viruses play significant roles in various ecosystems, influencing population dynamics and nutrient cycles.
Ecological Roles:
- Population Control: By infecting and often killing a fraction of a population, viruses can prevent overpopulation and resource depletion.
- Nutrient Cycling: Marine viruses, especially bacteriophages, are crucial in nutrient cycling in oceans, releasing nutrients back into the environment.
Research Findings: Recent studies have shown that viruses can influence the carbon cycle in oceans, affecting global climate patterns.
Virology and Sociology: The Social Impact of Viruses
Viral outbreaks influence societies, shaping behaviors, economies, and cultures.
Societal Impacts:
- Behavioral Changes: Outbreaks can lead to changes in societal behaviors, from simple hand hygiene practices to broader shifts like remote work adoption.
- Economic Impacts: Epidemics and pandemics can have significant economic consequences, affecting global trade, tourism, and labor markets.
Research Findings: The COVID-19 pandemic led to a global shift towards remote work, influencing urban planning, transportation, and workplace cultures.
Virology and Technology: Digital Tools in Virus Study
Modern technology, from advanced imaging techniques to artificial intelligence, is reshaping virology.
Technological Advancements:
- AI in Drug Discovery: Machine learning algorithms can analyze vast datasets to predict potential antiviral compounds.
- Digital Epidemiology: Mobile apps and online platforms can track disease spread in real-time, aiding in outbreak control.
Research Findings: AI platforms were instrumental in the rapid analysis of the SARS-CoV-2 genome, leading to quicker insights into its biology.
The Future of Virology: Innovations and Challenges
Personalized Antiviral Therapies
As we understand more about individual genetic makeup and its influence on disease susceptibility and treatment outcomes, there’s a growing interest in personalized medicine in virology.
Key Concepts:
- Genetic Profiling: By understanding an individual’s genetic makeup, treatments can be tailored for maximum efficacy and minimal side effects.
- Pharmacogenomics: This field studies how genes affect a person’s response to drugs. In the context of virology, it can help determine which antiviral drugs will be most effective for a particular individual.
Research Findings: Early studies on HIV treatments have shown that individuals with certain genetic markers respond better to specific antiretroviral drugs.
Synthetic Virology: Designing Viruses for Good
The field of synthetic biology is allowing scientists to design and construct viral particles for beneficial purposes.
Potential Applications:
- Gene Therapy: Custom-made viruses can be used to deliver therapeutic genes to individuals with genetic disorders.
- Cancer Treatment: Oncolytic viruses are designed to specifically target and kill cancer cells, offering a novel approach to cancer therapy.
Research Findings: Early clinical trials using oncolytic viruses have shown promise in treating certain types of cancers, especially when combined with other therapies.
Global Collaboration and Open Science
The rapid sharing of data, especially during outbreaks, is becoming increasingly important. Open science initiatives in virology aim to promote collaboration and accelerate discoveries.
Key Initiatives:
- Viral Sequence Databases: Platforms that allow researchers worldwide to upload and analyze viral genome sequences.
- Collaborative Research Networks: Global networks of virologists collaborating on specific challenges, sharing data, and resources.
Research Findings: During the COVID-19 pandemic, the rapid sharing of the SARS-CoV-2 genome sequence allowed researchers worldwide to start working on diagnostics, treatments, and vaccines almost immediately.
Challenges Ahead: Ethical and Biosecurity Concerns
As with any rapidly advancing field, virology faces challenges related to ethics and biosecurity.
Key Concerns:
- Dual-Use Research: As discussed earlier, certain types of research have potential misuse concerns.
- Equity in Treatment Access: Ensuring that advancements in virology benefit all, and not just a privileged few, is a significant challenge.
Research Findings: The global scramble for COVID-19 vaccines highlighted disparities in access, with wealthier nations securing more doses initially.
Advancements in Diagnostic Tools and Techniques
Rapid Diagnostic Tests (RDTs)
RDTs have revolutionized the field of virology, allowing for the quick detection of viruses in various settings, from sophisticated labs to remote field locations.
Key Features:
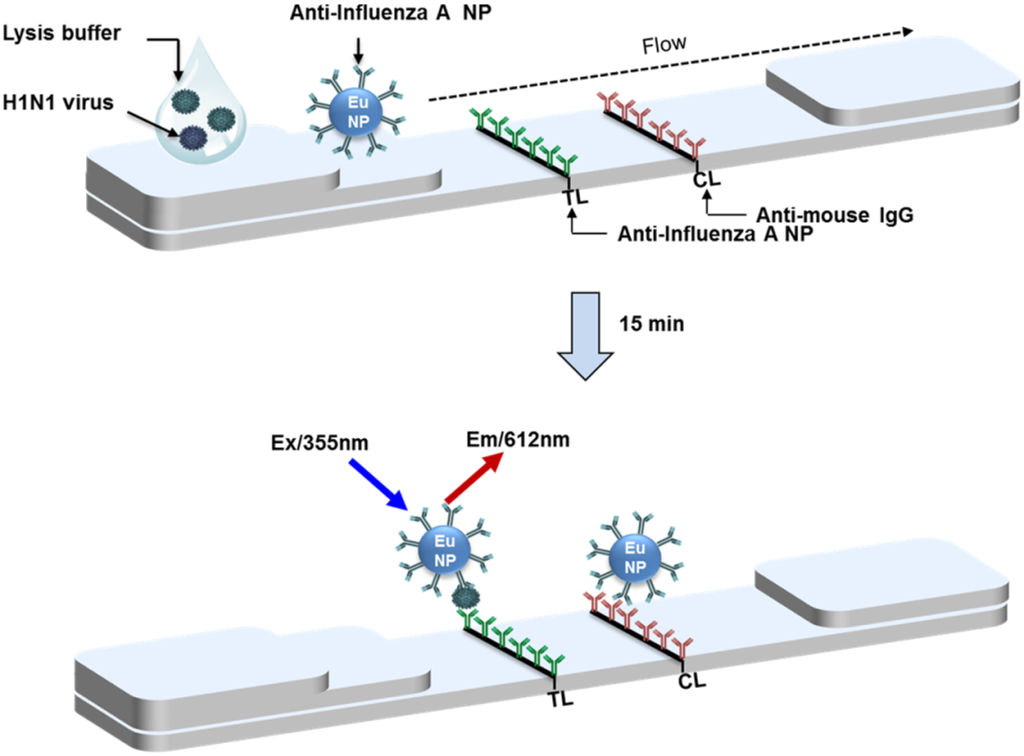
From: Clinical evaluation of rapid fluorescent diagnostic immunochromatographic test for influenza A virus (H1N1)
- Speed: Many RDTs can provide results in less than 30 minutes.
- Portability: Some RDTs are designed for use in field settings, requiring no specialized equipment.
Research Findings: The development of RDTs for diseases like HIV and malaria has significantly improved early detection and treatment initiation, especially in resource-limited settings.
Next-Generation Sequencing (NGS) in Virology
NGS allows for the rapid sequencing of entire viral genomes, providing insights into viral evolution, transmission, and drug resistance.
Applications:
- Outbreak Tracking: By sequencing viruses from different patients, researchers can track the spread and evolution of an outbreak in real-time.
- Vaccine Development: Understanding the genetic makeup of viruses can aid in the development of effective vaccines.
Research Findings: During the COVID-19 pandemic, NGS played a pivotal role in the rapid sequencing of the SARS-CoV-2 virus, allowing for global collaboration in research.
Digital Health Platforms and Diagnostics
The integration of digital technologies with diagnostics offers new possibilities for data collection, analysis, and patient management.
Key Innovations:
- Telemedicine: Remote consultations can guide individuals on when and where to get tested.
- Digital Tracking: Apps and platforms can notify individuals of potential exposure to viral diseases.
Research Findings: Digital contact tracing apps, introduced during the COVID-19 pandemic, showcased the potential (and challenges) of integrating digital technologies with public health efforts.
Point-of-Care (POC) Testing
POC tests are designed to be used directly at the point of patient care, providing immediate results and enabling timely clinical decisions.
Benefits:
- Immediate Results: Clinicians can make informed decisions without waiting for lab results.
- Decentralized Testing: Reduces the burden on centralized labs and speeds up large-scale testing efforts.
Research Findings: POC tests for diseases like influenza and strep throat have become standard in many clinics, improving patient management and reducing unnecessary antibiotic use.
Community Engagement and Public Health Campaigns
The Power of Grassroots Movements
Community-based initiatives play a pivotal role in disseminating accurate information, dispelling myths, and ensuring adherence to preventive measures during viral outbreaks.
Key Aspects:
- Local Leaders: Engaging community leaders can help in building trust and ensuring that health messages are culturally sensitive and relevant.
- Community Health Workers: These frontline workers often bridge the gap between health systems and communities, playing a crucial role in surveillance, education, and intervention.
Research Findings: In the fight against polio in various parts of the world, community engagement was instrumental in increasing vaccine acceptance and dispelling myths.
Harnessing the Power of Media in Public Health Campaigns
Media, from traditional outlets to social platforms, plays a significant role in shaping public perceptions and behaviors during viral outbreaks.
Strategies:
- Collaboration with Influencers: Partnering with popular figures can amplify public health messages and reach wider audiences.
- Interactive Campaigns: Using interactive tools, quizzes, and challenges can engage the public and make health education more impactful.
Research Findings: During the early days of the HIV/AIDS epidemic, media campaigns played a crucial role in educating the public about transmission routes and preventive measures.
Educational Programs in Schools
Integrating virology and public health education into school curriculums can equip the younger generation with the knowledge and skills to navigate future health challenges.
Key Components:
- Hands-on Workshops: Interactive sessions where students can engage in simulations, role-playing, and experiments related to virology.
- Digital Platforms: E-learning modules and apps that provide engaging content on viruses, their transmission, and prevention.
Research Findings: Schools that integrated health education into their curriculums saw a marked increase in students’ knowledge about diseases, leading to better hygiene practices and reduced absenteeism.
Public-Private Partnerships in Health Campaigns
Collaboration between governments, NGOs, and private entities can amplify the reach and impact of public health campaigns.
Benefits:
- Resource Mobilization: Private entities can provide financial and logistical support, enhancing the scale of health campaigns.
- Innovation: Collaboration can lead to innovative solutions, from tech platforms for disease surveillance to novel strategies for community engagement.
Research Findings: The Global Polio Eradication Initiative, a partnership between WHO, Rotary International, the CDC, and UNICEF, has been instrumental in reducing polio cases by over 99% since its inception.
The viral life cycle involves attachment to host cells, penetration, uncoating of the viral genome, replication within the host, assembly of new virions, and their release. DNA and RNA viruses employ diverse replication strategies. The host’s immune response, including innate and adaptive defenses, is critical in fighting viral infections. Retroviruses, like HIV, convert their RNA genome into DNA and integrate it into the host’s genome. Viral proteins, such as attachment proteins, facilitate host cell entry. The virus-host interaction is a complex, evolving relationship with implications for disease and treatment.
Disclaimer:
- “The information provided in this article is for educational purposes only and is based on current research and knowledge as of the date of publication. While efforts have been made to ensure accuracy, the field of virology is vast and ever-evolving. Readers are encouraged to consult primary sources and professionals in the field for the most up-to-date and comprehensive information.